|
|
|
|
1. Project Partner
Joined for a Better Environment
- Puerto Rico Water Resources and Environmental Research Institute
- University of Puerto Rico at Mayagüez
- United States Geological Survey
2. Researchers
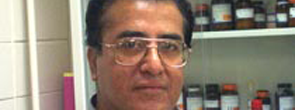
Oscar J Perales-Pérez Ph.D.
Department of Engineering Science and Materials
University of Puerto Rico
Mayagüez Campus
Felix R. Román-Velázquez Ph.D.
Department of Chemistry
University of Puerto Rico
Mayagüez Campus
3. Students

Boris Renteria
University of Puerto Rico - Mayagüez Campus
Doctoral Candidate : Civil Engineering
Environmental Engineering Program
Graduation Date: December 2021
Masters of Science in Engineering (2007)
University of Atlántico, Barranquilla - Colombia
Bachelor of Science in Mechanical Engineering (2001)
e-mail: boris.renteria@upr.edu
|
|
|
|
Nanotechnology-based wastewater treatment for reuse phase I
|
Duration: June 18 2019 – December 31st 2020
Sponsoring Agency: United States Geological Survey (USGS)
Project Strategy, Technical Assistance
and Training Approaches
1. Project Narrative
Industry consumes large amounts of water in steam generation, cooling, washing, sanitation, consumption,
irrigation, among others. According to Dieter, et al. [1], the 2015 US consumption of water was about 322
billion gallons per day (Bgal/d); 237 Bgal/d came from surface water and 84.7 Bgal/d from groundwater
sources. The industrial sector consumed an estimated 14.8 Bgal/d of which 12.1 came from surface water
sources and 2.7 from groundwaters. These demands of water come from the producers of food, paper, chemicals,
refined petroleum, and primary metals. These levels of water consumption give an idea of the volumes of
wastewater that will be discarded to the public sewer system and would mostly discharge to the rivers
and eventually to the sea.
At a local level, the Environmental and Natural Resources Department (DRNA, for its Spanish acronym)
revealed that Puerto Rico’s annual demands for 2005 were 21,246.2 Millions of gallons (MG) which will
reach 25,407.9 MG for agricultural purposes only in 2025 [2]. Regarding sanitary water generation in 2015,
it averaged 232 MG/day according to the Aqueducts and Sewers Authority (AAA) [3].
There is no concrete data on the amount of reusable water, however, it is known that there are initiatives
in private sectors that have implemented these practices in their industries. The regulations established
by the EPA and the regulation of the water quality standards of Puerto Rico and the Clean Water Act encourage
water reuse. However, there would be two factors that complicate the implementation of reuse projects: the
high initial costs of the facilities to process the water, and the low political priority.
The priority in water reuse processes is the reduction of the risk of the potential spread of diseases,
especially if sanitary water is used. Pathogenic microorganisms can exist in the water that is planned to
be reused for agricultural irrigation. Evidently, new sources of water for general reuse, particularly for
agricultural applications, became indispensable. Accordingly, the present research will investigate and
develop additional alternatives that will bring these waters suitable for future reuse. Recently, several works
about the use of nanostructured materials in water treatment have been developed. One approach is related
to the use of magnetic ferrites as the dispersed phase within polymer-based nanocomposites.
The synergistic co-existence of these two materials (magnetic and bactericidal properties of the magnetic
nanomaterials and the sorbent characteristics of the polymeric matrix) opens a new window of possibilities
for its application in biotechnology and environmental engineering. Although few reports suggested the
bactericidal capacity of magnetic nanoparticles, there is still a lack of systemic there is still a lack of systematic research about
the corresponding mechanisms and how the bactericidal capacity could be enhanced by proper control of the nanomaterial composition
and surface chemistry. In turn, the development of a net surface charge onto bacterium surface and the possibility of
tuning the surface charge of nanoscale ferrites just by suitable control of pH, or surface chemistry
(use of surfactants or other adsorbed species), opens the possibility of taking advantage of a strong electrostatic
interaction, i.e. nanoscale ferrites will attract oppositely charged bacterium and remove by the application of a moderate external magnetic field.
Based on the above considerations, the novelty of the present research relies on the use of bio-polymeric nanocomposites bearing magnetic ferrites as a bi-functional and environmentally friendly bacterium removal agent. As a bonus, the polymeric matrix (porous Calcium alginates beads or cellulose electrospun fibers, for instance) will also exhibit adsorption capacity for different types of inorganic and organic pollutants, commonly found in sanitary waters. The first phase of the research was focused on the nanocomposite synthesis and preliminary evaluation as a bacterium removal agent. This proposal will address the optimization of bacteria removal capacity of the selected nanocomposite and fabrication of a lab-scale prototype involving all stages to be considered in real operation.
Statement of regional or State water problem
Drought season in Puerto Rico is severe and has a great effect on water availability for all purposes, particularly for the irrigation of agricultural soils. Under critical drought conditions of the 2014-2016 drought in Puerto Rico, valuable drinking water was used to satisfy minimum agricultural needs and animal feeding [2]. A simple, cost-effective, and environmentally friendly water treatment for reuse will bring an environmentally innovative vision for the island and contribute to mitigating the effects of water scarcity. The application of reused water will directly impact the agricultural sector, which suffered losses of $34 million in the 2014-2015 drought [2].
The present project seeks to identify an alternative approach, involving a magnetic nanocomposite with the combined capacity to remove inorganic, organic and biological pollutants from water. The nanocomposite will be used to clean up sanitary waters processed in the wastewater treatment plants prior to the disinfection process and assess its quality to be reused rather than disposed to rivers and the ocean, which is the common practice in the Island. The use of nanoparticles provides an attractive and potentially cost-effective alternative compared to traditional methods of disinfection, such as the use of chlorine and ultraviolet light, since using biocompatible materials will not be conducive to the generation of toxic byproducts that may demand additional post-treatment stages.
Statement of results and benefits
The expected outcomes of this project can be summarized as follows: (a) Development of a novel magnetic nanocomposite with enhanced bactericide capacity and bacteria removal (due to surface chemical interactions between nanoparticles and targeted microorganisms) capability; (b) Development of an alternative approach to treat sanitary waters and enable its reuse for agricultural purposes (crops and soils irrigation); (c) Training of students in materials processing and bacterial removal and growth inhibition capacities protocols; (d) Potential generation of intellectual property.
The present proposal will take advantage of the preliminary results validated in phase I. Accordingly, the purpose of the phase-II is to maximize the bacteria removal capacity of the biopolymer-cobalt ferrite nanocomposite and determine the operational parameters in columnar magnetic removal tests in synthetic and actual water samples. For this latter purpose, a setup of continuous lab-scale magnetic removal of bacteria by the biopolymeric nanocomposite will be made and tested. The removal of heavy metal ions, phosphate, and nitrate species will also be evaluated. Samples generated by the Wastewater Treatment Plant in Mayagüez, Puerto Rico will be used for that purpose.
Nature, Scope and Objectives of the project
Nature and Scope
The proof-of-concept related to the electrostatic interaction between cobalt ferrite nanoparticles and E. coli, was achieved in the first phase of the project. This second phase of the project is focused on the preparation of Ca-alginate or cellulose fibers a matrix to host the cobalt ferrite nanoparticles. The resulting nanocomposites will be contacted to different bacteria populations at different bacteria/ferrite and bacteria/nanocomposite w/w ratios to determine the optimum conditions to fully remove the targeted bacteria. Selected bacteria will be the same as those commonly found in wastewaters. Magnetic ferrites will be used based on its antibacterial capacity as well as its suitability as a substrate for pathogen collection via electrostatic interactions. The biopolymers are also expected to exhibit adsorption capability for inorganic (i.e. heavy metals ions) and organic (solvents or others) contaminants. On this basis, the objectives of the present proposal are stated as follows:
Main Objective
Determine suitable operational parameters in columnar magnetic removal tests and determine the effect of co-existent ionic species in synthetic and actual wastewater samples.
Specific Goals
a. Determine the capability of ferrite surface modification to remove microbial pathogens by interfacial electrostatic interaction.
b. Determine the mechanisms involved with the bactericide capacity of the ferrites (for this purpose, the generation of Reactive Oxygen Species will be assessed using commercial kits).
c. Determine the Processing conditions to produce biopolymers for the formation of beads and electrospun fibers and the corresponding nanocomposite.
d. Design and build a lab-scale system to determine the efficiency of bacteria removal by the nanocomposite under continuous flow conditions
Timeline of Activities
The workable timetable for the phase-II work is summarized as follows:
Methods, procedures and facilities
Methodology
-
The water pollutant - The project will focus on the biological contaminant present in the effluents from municipal and industrial wastewater treatment plants, after the removal of suspended solids and before the disinfection stage.
-
Synthesis of Cobalt ferrite (CoFe₂O₂) nanoparticles – It will be based on the modified coprecipitation method developed by our group [4]. A 0.055M Co (II) and 0.11M Fe (III) are contacted with an excess of hydroxide ions under water boiling conditions. The precursor solid will go through a dehydration and atomic rearrangement to finally give the CoFe₂O₂. Different temperatures and time of synthesis will be evaluated to control the ferrite particle size in the 10nm - 30nm range.
-
Synthesis of the biopolymer matrix – The Ca-alginate beads- The matrix will be synthesized according to the protocol developed by our group [5], [6], [7], [8]. In short, a Na-alginate solution will be added dropwise to an aqueous CaCl2 aqueous solution under gently mixing conditions. The presence of Ca ions will promote the gelation of the Ca-alginate and final precipitation as micrometric beads. The size of the beads will be attempted by controlling the size of the drop of Na-alginate, which will be varied using syringes with different needle diameters. For the cellulose fibers, one gram of the microcrystalline powder will be dissolved in an 8.5% NaOH solution and then cooled to -20 °C. The frozen solution is left at room temperature and once it is thawed, an adequate amount of water is added, resulting in a clear and homogeneous cellulose solution [9].
-
Synthesis of the magnetic nanocomposite – The cobalt ferrite nanocrystals, at different weight/weight loads, will be ultrasonically dispersed in the Ca solution prior to the addition of the Na-alginate [7], [8]. Alternatively, CaCl2 will also be added dropwise into the Na-alginate solution. The immobilization of the ferrite nanoparticles (30nm in average diameter) inside the macroscopic polymeric matrix will enable their handling as required in a real application. The surface charge of the ferrite nanoparticles outside and inside the polymer matrix will be carried out by their surface functionalization using ionic surfactants or by simple adjustment of the water pH in the 5-8 range. As an alternative approach a polymer/ferrite core-shell arrangement will also be attempted by adding the ferrite suspension just before the gelation reaction ends. It will cause a large amount of ferrite to be deposited onto the matrix substrate, and hence, a larger number of active sites surface to destroy pathogens and remove them by electrostatic interactions. This composite can be prepared by polymerizing monomer droplets stabilized by magnetic particles in the emulsions [10].
- The use of an electrochemical manufacturing method will be selected to produce cellulose nanofibers combined with the nanoparticles. One of the routes to follow to achieve these multifunctional nanofibers is the addition of cobalt ferrite nanoparticles at a convenient stage during the process of developing the cellulose solution for electrospinning, with the purpose of promoting exposed surfaces of nanoparticles in the areas external fibers [11], [12], [13], [14].
Materials characterization adn bacteriacide capacity assessment
Structural and morphological analyses of produced nanostructures – They will be carried out by XRD, VSM, SQUID, FT-IR, SEM, and TEM techniques. The measurement of magnetization and coercivity will be undertaken in a Quantum Design XL-7 Magnetic Property Measurement System (MPMS), based on a Superconducting Quantum Interference Device (SQUID) and a magnetometer of the type VSM of Lake Shore.
Bacteriological tests – The ASTM E2180-07 (Standard Test Method for Determining the Activity of Incorporated Antimicrobial Agent (s) in Polymeric Materials or Hydrophilic) [15] will be followed. The colony-forming units will be calculated by multiplying the number of colonies by the dilution factor and the survival percentage will be used. The microorganism survival percentage will be used to evaluate the antimicrobial effect of particles, according to:
Preliminary results: Synthesis of Cobalt ferrite
Figure 1-a shows the XRD patterns of the ferrite samples synthesized after 30 minutes of reaction, under non-controlled flow rate conditions and intensive heating. All the peaks correspond to the ferrite cubic structure. The average crystallite size, estimated by the Scherrer's equation was 19 nm. Figure 1-b shows the magnetic hysterisis loop for the ferrite. A maximum magnetization of 7.2 emu/g and coercivity of 362 G, where achieved. The magnetic properties will be further enhanced after optimizing the system conditions.
Figure 1. a) XRD pattern, and b) Magnetization hysteresis loops for the as-synthesized CoFe₂O₂ nanoparticles. Evidently the modified co-precipitation method was successful to produce the desired magnetic material.
Facilites
The PIs host all the necessary facilities and resources to carry out the project and its associated goals and objectives.
- Analytical Laboratory for Foods, Soils and Environmental Chemistry (ALFSEC) - ALFSEC is located in the Chemistry building (laboratories Q-209, Q-215, and Q-279) under the direction of Dr. Félix R. Román, Co-PI of this proposal. ALFSEC is equipped with state-of-the-art instrumentation for the analysis and characterization of environmental samples, including the following: Agilent Technologies 7500 Inductive Coupled Plasma Mass Spectrometer (ICP-MS) equipped with cell reaction technology to reduce matrix interferences; Agilent Technologies 1100 High-Performance Liquid Chromatograph equipped with Bruker Daltonics Esquire 6000 Mass Spectrometer detector with electrospray, atmospheric pressure chemical ionization and photoionization sources, Agilent Technologies 1100 High-performance Liquid Chromatograph equipped with diode array and fluorescence detectors; Finnegan Polaris Gas Chromatography-Mass Spectrometry system equipped with electron impact and chemical ionization sources, Horiba Raman Microscope Xplora system, and Perkin Elmer IR System. Additional equipment includes a Mars Microwave Digestion-Extraction System, a Shimadzu 1800 UV-Visible spectrophotometer, SANYO incubators, QUEBEC Darkfield colony counters, centrifuges, -75 ºC Freezer, and other minor equipment.
- Materials Research Laboratory I - This 960 ft² laboratory is in the Stefani Building (Material Science and Engineering), Room S-311/312, and is outfitted with the instrumentation used for both instruction and research. Four main pieces of equipment are housed in this room. The first one, a Mettler Toledo 851eTGA/SDTA unit with 0-1,000 °C temperature range that has been recently upgraded with state-of-the-art software provided by the manufacturer. Second, a Siemens D500 X-ray diffractometer is used for conventional structural analysis. The unit, equipped with a CuKα source, possesses an automatic sample exchanger system for systematic analysis. To complement phase and structural studies, this laboratory facility is furnished with a 6 ft. Labconco laminar flow fume hood with an external exhaust system and audiovisual capabilities to support instructional modules.
- The NANOmaterials Processing Laboratory - This laboratory occupies 1,050 ft² and is located in the Stefani Building, Room S-313. The laboratory is outfitted with a 74110 Lakeshore VSM magnetometer capable of generating magnetic fields up to 3.1 Tesla and equipped for high-temperature measurements (1,000 ºC). A second magnetometer has been recently acquired, which is a Quantum Design MPMSXL Superconducting Quantum Interference Device. The laboratory also houses two optical microscopes equipped with digital cameras: a Nikon Epiphot 200 inverted microscope (with a 100x optical lens and Nomarskifilters) and a Nikon SMZ1500 stereozoom one. The lab also has a TA Instruments Q20 differential scanning calorimeter, a Beckman DU800 UV-Visible Spectrophotometer, a Shimadzu IRAffinity-1 Infrared Spectrometer, a Horiba SA – 9600 series Surface Area Analyzer, and a Shimadzu RF-5301 Spectrofluorometer. A Malvern Zetasizer Nano-ZS90 is available for particle size measuring using Dynamic Light Scattering, also with the ability to measure zeta potential and electrophoretic mobility using Laser Doppler Microelectrophoresis.
This laboratory also comprises a 900 ft² space intended for basic manufacturing and advanced synthesis of materials. Among the instrumentation relevant for this proposal, there is a Cee 200 Spin-coater system from Brewer Science, Inc., a Mercury-Xenon 200W-UV lamp from Oriel Instruments, Inc., and a Thermo Fisher vacuum oven. Three dispersing tools available in the lab are: a high spindle speed homogenizer (KA T18 with S18N-19G dispersing tool), a low spindle speed Labmill–8000, and a Cole Parmer ultrasonic processor. Additional equipment for material synthesis and/or processing include: a 4575 model HP/HT Pressure Reactor from Parr Instruments Company, a 1,100 °C Vacuum Chamber Furnace (7.5"IDx 13"L, 7.6 Liter) with 30 Segments Programmable Temperature Controller - VBF-1200X-H8, and a Microwave Accelerator Reactor System, Model MARS 6 from CEM Corporation is also available for synthesis experiments.
Related Research
Ferrites for water disinfection
Among the various ferrite materials for environmental applications, cobalt ferrite (CoFe₂O₂) is a very promising candidate; however, its applicability has been scarcely investigated. This ferrite exhibits excellent chemical and thermal stability [17],[18], electrical insulation, wear resistance [19], good mechanical hardness and adequate magnetic susceptibility and coercivity, compared to magnetite Fe3O4. These combined properties facilitate large interactions and promote more rapid chemical reactions [20],[21],[22]. Its composition and microstructural properties are dependent on the synthesis process [10][17], a factor that enables their precise control of size, shape, and dispersion in aqueous and polymeric matrices [10].
Polymer-magnetic nanoparticle composites –
Very preliminary tests showed favorable results against Gram-positive bacteria and Gram-negative bacteria, e.g. Escherichia coli (E. coli), Staphylococcus aureus (S. aureus), S. cerevisiae, candida species, C. parapsilosis, C. krusei, and C. albicans [10],[22],[23]. Interestingly, the size of the ferrites played an important role in the efficiency of their antimicrobial capacity [24]. Available studies have reported a certain size-dependence of antimicrobial capacity in nanoparticles in the 8 nm - 50 nm range [18], [24], [25]. The studies carried out by Kooti et al [15], [23], [24], offer a guide for the evaluation of the bactericidal property of nanocomposites based on cobalt ferrites, against specific bacteria representing the groups Gram-negative and Gram-positive. These biocompatible nanocomposites can also be functionalized with bactericide species (silver or commercial antibiotics) to enhance their bactericide effect [17], [27], [28].
The mechanisms that induce the destruction of the bacteria are still under discussion. Some reports suggested the generation of reactive oxygen species (ROS) after the nanoparticle invades the internal part of the cell; a route that could be promoted by electrostatic interaction between the nanoparticles surface and the cell wall [10], [30]. Once inside the cell, the nanoparticles would cause degradation and lysis of the cytoplasm. In other cases, the particles may adhere to the microorganism membrane, which causes an elongation in the phase of retardation of the growth cycle, and in this way, the generation time of organisms is increased so that each organism takes more time to complete the cell division [13].
Based on the above considerations, the present proposal will address the viability in increasing the bactericide capacity of magnetic nanoparticles by modification and tuning of their composition (pure and doped Cobalt ferrite), size at the nanoscale, surface chemistry (functionalization with antimicrobial species) in absence and under the effect of a variable external magnetic field. Fecal Streptococci and E. Coli will be selected as targeted pathogens. Complementary, the sorption capacity of the Ca-alginate matrix (beads) to remove inorganic (metals ions, nitrates) usually reported in polluted waters, will also be determined by ICP and suitable analytical techniques available at our facilities. The bacteria removal by electrostatic interaction will be dependent on the differences in the zeta potential of bath the ferrite phase and the microorganism. In other words, the larger the discrepancy in zeta potential, the higher the efficiency of bacteria removal by application of a magnetic field to the ferrite and nanocomposites.
To the best of our knowledge, this research will be the first one to carry out systematic research on the bacteria removal capacity of as-synthesized and surface functionalized ferrite (nanopowders and bipolymeric nanocomposites). The cobalt ferrite particles will be functionalized with chemical groups (e.g. amines) to enhance the difference between the zeta potential of the materials and the microorganisms.
Training Potential
One graduate (civil engineering doctorate program) and two undergraduate students (chemical engineering), the later enrolled in the Undergraduate Research course offered by Román (Co-PI) and Perales (PI), will receive hands-on training on the synthesis, surface functionalization and characterization (structural, chemical, morphological, magnetic) of the materials involved with the research. Their research experience will be enriched by their involvement in the learning of the biological protocols to determine the bactericide capacity of the nanocomposites. As a complement, they will reinforce their soft - skills by participating in oral and poster presentations and preparation of manuscripts and research proposals to assure the project sustainability beyond the funding period.
References
-
División Monitoreo del Plan de Aguas, “Informe sobre la Sequía de 2014-2016 en Puerto Rico,”
Departamento de Recursos Naturales y Ambientales de Puerto Rico, 2016.
-
F. Quiñones and R. Guerrero, “Plan de reuso de aguas usadas de Puerto Rico,” Oficina del Plan de
Agua, Departamento de Recursos Naturales y Ambientales de Puerto Rico, 2004.
-
D. Sánchez-Rivera, O. Perales-Pérez, and F. R. Román, “Removal of inorganic arsenic oxyanions
using Ca-Fe(III) alginate beads,” Desalin. Water Treat., vol. 51, no. 10–12, pp. 2162–2169, 2013.
-
J. López-Morales, D. Sánchez-Rivera, T. Luna-Pineda, O. Perales-Pérez, and F. Román-Velázquez,
“Entrapment of Tyre Crumb Rubber in Calcium-Alginate Beads for Triclosan Removal,” Adsorpt. Sci. Technol., vol. 31, no. 10, pp. 931–942, 2013.
-
R. Pineda, T., L., Rivera, M., O., Pérez, O., P., & Velázquez, F., “Removal of Arsenic from Aqueous
Solutions with Alginate Based-Magnetic Nanocomposites University of Puerto Rico at Mayagüez ; Department of Engineering Science & Materials,” Clean Technol., vol. 2, pp. 261–264, 2009.
-
T. Luna-Pineda, M. Ortiz-Rivera, O. Perales-Pérez, and F. Román-Velázquez, “Synthesis and
Characterization of Alginate Based-Magnetic Nanocomposites,” NSTI-Nanotech, vol. 2, pp. 261–264, 2009.
-
R. Žalnėravičius, A. Paškevičius, M. Kurtinaitiene, and A. Jagminas, “Size-dependent antimicrobial
properties of the cobalt ferrite nanoparticles,” J. Nanoparticle Res., vol. 18, p. 10, 2016. .
-
E. Eriksson, K. Auffarth, M. Henze, and A. Ledin, “Characteristics of grey wastewater,” Urban
Water, vol. 4, no. 1, pp. 85–104, 2002.
-
Y. Xu, C. Li, X. Zhu, W. E. Huang, and D. Zhan, “Application of magnetic nanoparticles in drinking
water purification,” Environ. Eng. Manag. J., vol. 13, no. 8, pp. 2023–2029, 2014.
-
N. Sanpo, C. Wen, C. C. Berndt, and J. Wang, “Antibacterial properties of spinel ferrite
nanoparticles,” in Microbial pathogens and strategies for combating them: science, technology and education, Microbiolo., A. Méndez-Vilas, Ed. FORMATEX, 2013, pp. 239–250.
-
M. Kooti, P. Kharazi, and H. Motamedi, “Preparation, characterization, and antibacterial activity of
CoFe₂O₂/polyaniline/Ag nanocomposite,” J. Taiwan Inst. Chem. Eng., vol. 45, pp. 2698–2704, 2014.
-
R. S. Ayers and D. W. Westcot, “Water Quality for Agriculture,” FAO of the UNITED NATIONS,
1985. [Online]. Available: http://www.fao.org/docrep/003/T0234E/ T0234E07.htm. [Accessed: 31-Jan-2017].
-
R. A. Corbitt, STANDARD HANDBOOK OF ENVIRONMENTAL ENGINEERING, Second edi. 1985.
-
N. Sanpo, J. Wang, and C. C. Berndt, “Influence of chelating agents on the microstructure and
antibacterial property of cobalt ferrite nanopowders,” J. Aust. Ceram. Soc., vol. 49, no. 1, pp. 84–91, 2013.
-
M. Kooti, S. Gharineh, M. Mehrkhah, A. Shaker, and H. Motamedi, “Preparation and antibacterial
activity of CoFe₂O₂/SiO₂/Ag composite impregnated with streptomycin,” Chem. Eng. J., vol. 259, pp. 34–42, 2015.
-
Stone, W. W., Gilliom, R. J., and Martin, J. D., 2014. An Overview Comparing Results from Two
Decades of Monitoring for Pesticides in the Nation’s Streams and Rivers, 1992–2001 and 2002–2011, , U.S. Geological Survey Scientific Investigations Report 2014–5154, 23 p., http://dx.doi.org/10.3133/sir20145154.
-
S. Velho-Pereira et al., “Antibacterial action of doped CoFe₂O₂ nanocrystals on multidrug
resistant bacterial strains,” Mater. Sci. Eng. C, vol. 52, pp. 282–287, 2015.
-
A. Pratt, “Environmental Applications of Magnetic Nanoparticles,” in Nanomagnetism:
Fundamentals and Applications, 1st ed., vol. Volume 6, Elsevier Ltd., 2014, pp. 259–307.
-
B. Ding, J. Kim, E. Kimura, and S. Shiratori, “Layer-by-layer structured films of TiOࠢ
nanoparticles and poly (acrylic acid) on electrospun nanofibres,” Nanotechnology, vol. 15, no. 8, p. 913, 2004.
-
M. Kooti, S. Saiahi, and H. Motamedi, “Fabrication of silver-coated cobalt ferrite nanocomposit
and the study of its antibacterial activity,” J. Magn. Magn. Mater., vol. 333, pp. 138–143, 2013.
-
A. Hathout et al., “Synthesis and characterization of cobalt ferrites nanoparticles with cytotoxic
and antimicrobial properties,” J. Appl. Pharm. Sci., vol. 7, no. 01, pp. 086–092, 2017.
-
P. B. Koli and K. H. Kapadnis, “Synthesis, Characterization & Antimicrobial Activity of Mixed Metal
Oxides of Iron Cobalt Nickel and Zinc,” Int. J. Chem. Phys. Sci., vol. 4, pp. 357–360, 2015.
-
N. Sanpo, C. C. Berndt, and J. Wang, “Microstructural and antibacterial properties of zinc-
substituted cobalt ferrite nanopowders synthesized by sol-gel methods,” J. Appl. Phys., vol. 112, no. 8, 2012.
-
J. Liu, C. Vipulanandan, T. F. Cooper, and G. Vipulanandan, “Effects of Fe nanoparticles on
bacterial growth and biosurfactant production,” J. Nanoparticle Res., vol. 15, no. 1, 2013.
-
N. Sanpo, C. C. Berndt, C. Wen, and J. Wang, “Transition metal-substituted cobalt ferritee
nanoparticles for biomedical applications.,” Acta Biomater., vol. 9, pp. 5830–5837, 2013.
|
|