|
|
|
|
1. Project Partner
Joined for a Better Environment
- Puerto Rico Water Resources and Environmental Research Institute
- University of Puerto Rico at Mayagüez
- United States Geological Survey
2. Researchers
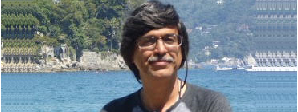
Walter Silva Araya, Ph.D.
Professor Civil Engineering and Surveying Department
University of Puerto Rico
Mayagüez Campus walter.silva2@upr.edu
|
|
|
|
Validation and demonstration of Vegetation Manning's n values for Retardance Index in the Caribbean Area, to Cover Waterways and Open Channels, and Reduce Risk of Erosion.
|
Duration: March 1, 2020 to December 31, 2021
Sponsoring Agency: United States Geological Survey (USGS)
Project Strategy, Technical Assistance
and Training Approaches
1. Project Narrative
Retardance and vegetative channel design
Channels with vegetative linings have been used for agricultural drainage for many years [1]. Well-designed vegetative channels convey runoff preventing flooding without causing erosion, protect the soil, avoid formation of gullies, improve water quality and reduce the generation of sediments [2]. They are a cost-effective way of erosion control in roads and highway projects [3], [4]. They are also used for critical areas such as levees, pond banks and gullies in agricultural fields.
Two main considerations are vital for good channel design: flow resistance and stability. Flow resistance is related to friction and form stresses generated between water and vegetative cover and, stability refers to prevention of channel degradation due to erosion.
Fulfillment of both conditions in grass lining is a challenging problem because grass is flexible and bends according to the flow conditions (Sturm, 2010). The presence of vegetation in waterways generates turbulence and, consequently, dissipation of energy and flow retardance [5]. Flow resistance coefficients will not remain constant for the case of grass boundary. For grass-lined channels the drag force on the vegetal elements dominates resistance to flow. If the depth of flow is less than the deflected grass height, velocity is mainly dependent on density of vegetation, spacing, type and quality. When vegetation is overtopped the vegetal elements tend to align with the flow and waving action is present. Increase in flow depth results in less influence from the bottom roughness and flow resistance decreases. The number and length of stems seems to have a major influence under these conditions. For large flows the depth of water is much higher than the deflected height of the vegetation, the influence of vegetation is reduced drastically and the flow resistance due to grass remains constant. The size and bulk of vegetation near the bed dominates the resistance. Flows in the intermediate zone are important for erosion and stability. Very low flows are not able to generate significant erosion, and extremely high flows are not frequent [6]. Presently there is a need for resistance relations for very low and very high flows over vegetative channels.
As early as 1949, Ree and Palmer [7] found that the Manning’s coefficient of resistance (n) can be expressed as a function of the product of the mean flow velocity (V) and the hydraulic radius (R). The hydraulic radius is the ratio of the wetted area (A) divided by the wetted perimeter (P) of the channel. A series of experiments developed between 1937 and 1953 by the Soil Conservation Service (SCS) allowed development of the first empirical correlations between retardance and flow conditions [6]. Those experiments arrived at a classification of species according to their degree of retardance [1]. The degree of retardance depends on the species, percent of cover and vegetation height; therefore, the classification of degree of vegetal retardance is based on the kind of vegetation and the growth stage. Five retardance classes were proposed after a series of experiments conducted by the NRCS (previously known as the Soil Conservation Service). Classes were named A, B, C, D and E according to the degree of retardance. Retardance class A refers to Very High resistance. The degree of resistance diminishes as the class moves from A to E. In general, taller and abundant vegetation corresponds to retardance A and B; while, shorter and scarce vegetation refers to C, D and E. Retardance also varies with degree of bending which correlates with the flow velocity and depth. Table 1 shows the classification of degree of retardance for grasses tested by the NRCS and published in 1954.
Manning’s equation is the most used formula to estimate channel capacity based on the channel geometry and the resistance. The Manning’s equation is given as [8]:
Where V is the average flow velocity, R is the hydraulic radius, S is the channel bottom slope, C is a unit factor equal to 1.0 for S.I units or 1.486 for E.S. units and, n is a resistance coefficient. NRCS found that Manning’s n varies over a wide range of grass conditions, species, channel geometry, channel slope and depth of flow. In addition, it was discovered that the value of Manning’s n coefficient is related with the product of the mean velocity, V, and the hydraulic radius, R. Consequently, a relation of n vs the product VR was obtained for different retardance classes. The classical relationship between the product VR and Manning’s n is given in Figure 1.
An important contribution to the understanding and applicability of the n-VR method was Temple’s synthesis of the family of retardance curves into one non-dimensional equation to represent the values of the Manning’s coefficient in terms of the product VR and a given “curve index”. This relation provides a mathematical formulation to reproduce the n-VR curves shown in Figure 1; as well as, other intermediate values not shown in this figure [9]. The retardance curve index depends on the stem length and the vegetation cover density. The Soil Conservation Service (previous name for NRCS) associated the curve index with the Retardance Class [6].
Recent advances in Vegetative Channel Design
Research on vegetative channels has made significant progress since the retardance classification was proposed; however, retardance classification continues to be a design parameter in vegetative channel design. Two major steps to improve vegetative channel design were the use of the Permissible Tractive Force instead of the Permissible Velocity as the design criterion [10] [11] and, the incorporation of biomechanical properties and their relation with the determination of the retardance classification, promoted mainly by Kouwen [12] [13] [14] [15] [4]. The main biomechanical properties of vegetation proposed have been the longitudinal modulus of elasticity, E, the moment of inertia, I, of the plant stems and, the vegetation concentration, M. The product EI is the bending stiffness. However, the correlations obtained were derived from experiments using artificial vegetation with a constant value of stiffness and pre-determined patterns. Carolo et al. (2005), showed that Kouwen’s method systematically overestimates the flow resistance and that the overestimation increases with concentration of vegetal cover. These authors proposed a new resistance law which includes a Reynold’s number parameter using the friction velocity and the bent vegetation height. An important result from this research was that the n-VR plots, corresponds to the expected behaviour of plastic vegetation. Stephan and Gutknecht [16] studied the influence of roughness resistance due to submerged macrophytes in a laboratory flume. These plants are flexible and have different behaviour depending on the flow conditions. Similar to grass cover, the deflected plant height is an important parameter for describing the influence of roughness and the hydraulic resistance; however, the wavy motion of the plants does not increase hydraulic resistance.
Several studies have been made in laboratory flumes to study the effect of vegetation on flow resistances. According to Jarvela [17], large variations in the friction factor with the relative roughness, defined as the ratio of the deflected height of the vegetation and the flow depth, were observed; even when combined with stiffer leafless willows. The roughness characteristics in the presence of vegetation is a combination of shape, size, arrangement, and concentration of the elements [18]. Evaluation of flow resistance for flexible vegetation requires consideration of the height of the bent vegetation for different hydraulic conditions and the influence of vegetation concentration [18]. Kouwen and Unny [19] suggested this behavior for grasses. The previous authors did not use the retardance class as a parameter in their research. More recently, other authors proposed a relation of Manning’s n with Froude number [20]; however, their data is limited and was only tested with relatively small discharges (< 0.06 m3/s). Research on vegetative waterways continues gaining importance by the fact that vegetative cover in channels and ditches helps to reduce transport of pollutants into rivers and creeks, reducing environmental impacts [21]. Limitations in experimental channel size and slope; as well as, pumping capacity, make most of the proposed methods of limited use for general agricultural applications; therefore, besides the attempts to provide alternative methods and convergence difficuties when solving using computer methods, the n-VR method continues to be the preferred design method [22].
Statement of regional or State water problem
Retardance Classes for Vegetative Cover in the Caribbean
The USDA-NRCS Caribbean Area identified sixteen (16) species as vegetative lining for waterways in Puerto Rico [2] and proposed a classification according to the degree of retardance. However, according to SCS-TP-61 [1], the relations accepted by NRCS were developed “primarily for use in the Western Gulf Region”; but, the results were recommended for general use in the Caribbean region. Presently, the retardance classification for species in Puerto Rico has not been validated. Retardance was also associated with soil texture, water velocity and vegetation condition. These relationships are summarized in Table 2.
Grass Species for Erosion Protection of Waterways in the Caribbean
Weeds or grasses can be found worldwide, and are one of the largest ecosystems in the world. They play a vital role as food and forage, energy, and wildlife habitat; as well as, provide carbon and water storage [23]. Grassed waterways are the most recommended practice for erosion prevention due to runoff events, protection and improvement of water quality, reduction of gully erosion and increase in slope stability [24] [25].
During the 1990’s grasslands cover in Puerto Rico increased mostly by reducing woodlands. Figure 1 presents land categories from Puerto Rico [26]. Pastures are concentrated in the East Region and on the coastal areas. According to the USGS, grassland covers approximately 41 % of the land area in eastern Puerto Rico; however, this percentage includes dry and moist pasture and emergent and seasonally flooded herbaceous wetlands [27].
Forage production in Puerto Rico includes a mixture of tropical species, some available in southern Florida and other native species. In the humid northern region predominant species are Pangola grass (Digitaria eriantha), guineagrass (Panicum maximum), and stargrass (Cynodon nlemfuensis). In the dryer areas the most common species are buffelgrass (Pennicetum ciliare), and bluestems (Dichantium sp.) [28]. Following is a brief description of six (6) grass species available in Puerto Rico and recommended as vegetative cover for erosion protection of waterways. Information was obtained from Más and García (2006) [29] and from USDA-EQB, 2005 [2].
1.Cynodon nlemfuensis is a perennial species that was imported from Africa to Puerto Rico in 1957. It has long stolons, grows fast and covers the soil with a dense sod. It reaches between 3 and 3.5 feet tall and is tolerant to droughts and floods.
2.Digitaria eriantha is known as Pangola grass, because it was imported from Pangola River in Africa. It grows rapidly and extends to produce a dense cover. It resists floods and droughts of short duration. It is a good choice for soil erosion protection and reaches more than 1.5 ft tall.
3. Dichantium annulatum, known as Pajón or Railroad-track grass is another grass recommended for erosion protection. It is abundant in the south coast. It adapt well to dry weather and tolerates relatively high salinity. Better erosion protection is achieved when it grows more than 20 inches tall.
4. Panicum maximum is also known as Urochloa maxima and it is original from Africa. It grows in arid regions, and does not tolerate floods.
5. Pennicetum ciliare is known as Buffel Grass. It also comes from Africa and is preferred for arid and semi-arid zones. Some variaties are found in kast zones between Guayanilla and Ponce. It is used in the south and southeast coast of Puerto Rico. It growths up to 2 ft.
6. Paspalum notatum is known as Bahía Grass. This is another species resistant to drought and able to survive in a broad variety of soils from sandy to clay. It is found in coastal areas [30].
This project will validate retardance classification and estimation of Manning’s “n” values. Four (4) species were identified as potential candidates for laboratory and field validation. Namely Pangola Grass, Bahía Grass, Buffel Grass and Star Grass. This project will compare retardance classification, Manning’s n values, plant conditions and channel flow variables. Caribbean NRCS will have the technical information needed for implementation of reliable waterway design using local grass species. Results will be useful for protecting ponds, levees, highway and other construction projects in preparation of effective Sediment and Erosion Control Plans (S&EC).
Nature, Scope and Objectives of the proposal
Nature
The objective of this proposal is to validate and demonstrate local vegetation-Manning’s “n” values for Retardance Index, in the Caribbean Area, to cover waterways and open channels and reduce risk of erosion.
This project will validate retardance classification and estimation of Manning’s “n” values. Four (4) species were identified as potential candidates for laboratory and field validation. Namely Pangola Grass, Bahía Grass, Buffel Grass and Star Grass. This project will compare retardance classification, Manning’s n values, plant conditions and channel flow variables. Caribbean NRCS will have the technical information needed for implementation of reliable waterway design using local grass species. Results will be useful for protecting ponds, levees, highway and other construction projects in preparation of effective Sediment and Erosion Control Plans (S&EC).
Project Methods
The methodology proposed combines advances in hydraulic engineering, plant bioengineering and controlled experiments, with well-known correlations for retardance classifications. These proven techniques guarantee a successful delivery of products.
1) Selection of Species
The proposed species are: Digitaria eriantha (Pangola grass) Paspalum notatum (Bahia Grass) Pennicetum ciliare (Buffelgrass) and ichantium annulatum (Pajón). This set of species covers all retardance classes from A to E, depending on the percent of cover and the average height.
Lab Experimental Program
2) Experimental design
Hydraulics of vegetative channels is a complex program. Detailed experiment preparation is required. The main reasons for this include:
-
Lack of scaling factors: Vegetation cannot be modeled at small scales because each plant is unique and real plant sizes must be used. Many experiments available in the literature were done using artificial rigid or flexible elements simulating grass-like behavior. However, researchers have shown that artificial elements do not reproduce the behavior of real vegetation [18], [22].
-
Range of values in n-VR curves: Experiments performed in fixed slope flumes and/or narrow flumes are not able to reproduce the combination of velocities and hydraulic radius expected in real waterways used for agricultural purposes. Those results cannot be used for validation of the n-VR because they are out of scale. Their purpose is usually to develop other relationships in terms of Froude number, Reynolds number or particular applications to bioengineering.
-
Risk of failure due to stability: The flow velocity must be controlled to avoid excessive shear stress to cause soil erosion and remove plants from the bottom. Therefore, maximum velocity should be kept below the value that exceeds maximum permissible shear stress.
A new flume will be built at the Department of Civil and Surveying Engineering of the University of Puerto Rico, Mayaguez. The channel will be designed specifically to study vegetative waterways accounting for the factors described in the previous paragraph. Figure 2 shows a schematic of the proposed channel. The channel will have at total length of 10 m (33 feet) and will be 1.05 m (3 ft) wide. It will be 1.05 m tall (3.0 ft) and will be supported over a pivot such that the slope can be changed. It will be built mostly on treated wood and sealed with fiberglass resin and impermeable paint. The walls on one side will be made of plexiglass for visibility. There will be an adjustable weir-gate at the downstream end to achieve desirable flow depths for specified VR values (V = mean velocity, R = hydraulic radius).
Water will be pumped from an existing pumping well located on the south side of the Department of Civil Engineering building. The facility has a multistage submerged pump. The pump will be connected to the new flume by a pipe system. Instrumentation necessary includes an Acoustic Doppler Velocimeter to obtain velocity profiles across the measurement section. This is required to avoid wall effects. Average velocity at the measurement section will be computed from the velocity profile and corroborated by a flow meter located along the pumping pipe. A control valve will be installed to regulate discharge (see Figures 2 and 3) and water depth in the flume will be measured using point-gages. The flume dimensions will be such that plant species could be inserted into the flume using modular trays accommodated side by side to form a testing reach. This will prevent perturbation of soil and roots and will allow more control of cover density and plants selection. Care will be taken to avoid measurements near the trays connections.
b) Experimental Validation Methodology
1. Channel slope and water depth will be established. The downstream gate will be adjusted for the desired water depth and the ADV will be located at the precise measurement section.
2. Plant properties will be measured from trays before placing in the flume. Properties include stem height, average diameter, density, soil gradation and soil specific gravity.
3. Trays containing the same grass species, size and density will be carefully placed at the channel bottom along the experimental reach, side by side.
4. The flume will be slowly filled with water to allow soil saturation and plants settlement.
5. Flow velocity will be incremented slowly by continuously providing water from the pump system until the desired discharge is obtained.
6. Time for establishment of steady-state flow conditions will be provided.
7. Measurements of flow, vegetation bent conditions, and velocity profiles will be obtained.
8. The slope of the energy grade line will be obtained by using precision water level gauges.
9. The previous information will then be used to obtain the shear stress on the plants and the roughness coefficient for Manning's equation (n). The average flow velocity and the hydraulic radius will also be measured.
10. Values of n versus product VR will be plotted for each grass species and vegetative conditions. The n-VR plot will be superimposed over the retardance classification curves to validate the NRCS relation.
Water flow velocity and shear stresses are related to the channel slope and water depth; therefore, both parameters (slope and depth) will be modified during the experiments. Experimental results should cover a range of product VR for different retardance classes. The duration of each experiment will be determined on a case-by-case basis, depending on the plants conditions and soil erosion potential (stability) observed during the experimental run.
b) Experimental Design
1. Survey and site preparation: A detailed survey of approximately a 200-foot-long portion of the test site at the UPR-M Civil Engineering and Surveying Complex will be performed. The site will be graded to a predetermined constant slope and channel shape. The drainage direction is already naturally determined and a parabolic like cross section already exists (See Figure 5). Once the ditch shape is determined, the site will be limited by using a fence for preservation of the experimental field.
2. Soil characterization: The area will be characterized using hydrologic, hydraulic and soil conditions. Precipitation, evaporation, infiltration capacity of the soils, soil fertility and soil classification will be obtained from existing equipment at the CE Environmental Laboratory.
3. Planting: The site will be planted with grass using one of the selected species. A planting plan and essential treatment will be prepared in consultation with agro-consultants from Plantas de Puerto Rico and assistance from UPR-M Agriculture Extension Division.
4. Measurements and monitoring: Grass density, biomechanical properties and height along different growth stages will be obtained. Experiments will be performed at different vegetation heights to obtain different retardance resistances. The experimental procedure is described next:
- a. Water will be supplied from a pumping well located near the site. The pumping well belongs to UPR-M and is used for graduate research in groundwater flow.
- b. Water discharge will be measured using a triangular (or rectangular) weir located at the downstream end. The weir will be used as control section.
- c. Water depths will be measured at four points along the waterway to determine water surface profile.
- d. Flow depths will be used to determine wetted perimeter, area, top width, hydraulic depth and hydraulic radius (R).
- e. Flow average velocity (V) will be obtained dividing flow discharge by channel area.
- f. Measured water discharge and water profiles will be used to determine the coefficient of Manning and the Retardance Class for the channel vegetative conditions.
- g. Values obtained from Step f will be used to validate the n-VR NRCS plots.
- h. The experiment will be repeated for three different grass species.
Outreach Program
The outreach and education program will consist of the following parts:
- Demonstration and seminar on vegetal cover impact for erosion control under different flow and plants submergence conditions
A half-day seminar and demonstration will be organized at the experimental site. Both, flume, and field experiments will be showed. The transparence of the plexiglass wall incorporated in the test flume is an excellent visual resource for observing these conditions. Visualization of plants behavior under partial submergence, total submergence and deep-water submergence will be presented. Data collection and processing; as well as calculation of the Retardance Index and Manning’s coefficient will be demonstrated as part of this activity.
Besides demonstration, the seminar will include a video presentation on the experimental program developed during the project duration, and a conference on fundamental concepts on water flow over submerged vegetation and the importance of vegetative waterways as green engineering and erosion control methods. Farmers and professionals in agricultural and environmental fields, personnel from environmental agencies (USDA-NRCS, DRNA, EQB), the Institute of Environmental Engineers of the College of Engineers and Surveyors of Puerto Rico (CIAPR), the Puerto Rico Wastewater and Environment Association (PRW&EA), faculty members of UPRM and the Agriculture Extension Services of UPR, and students from agriculture, agricultural engineering, environmental engineering and water resources engineering will be invited to this activity. This activity will be at the Civil Engineering and Surveying Department.
- Demonstration and seminar on vegetal cover impact for erosion control under different flow and plants submergence conditions
An illustrated guide showing local grass types, grass and soil conditions, and representative Retardance Index and Manning’s n values will be published. Fully colored photos and visuals collected during the project development will be combined with experimental results to make an easy-to-use attractive guide. This guide will assist Caribbean NRCS in selection of important parameters for practical applications on design and analysis of grassed waterways in agriculture. Moreover, this product will have expanded use for erosion protection of ponds, levees and construction projects in the Caribbean.
- Presentation of project results in social media
The project will help farmers, agronomists and engineers in application of vegetative covers as erosion protection and sediment control measures. Therefore, expanding results into social media will make it available to a broad audience. A series of You-Tube videos will be prepared and uploaded to communicate results to a broader audience. The website of the Puerto Rico Water Resources and Environmental Research Institute will be used to keep audience informed of the project progress and to publish project results.
- Impact on future professionals
This project will be located at the Mayaguez Campus of UPR. It will be a unique opportunity to show experiments and technology to undergraduate and graduate students pursuing careers in agriculture, agricultural engineering and civil engineering. The PI will invite members from the Faculty of Agriculture and Civil Engineering to visit with their students the experimental facilities. A demonstration will be done during these visits. At least 120 students are expected to be impacted per year, due to the strategic location inside the UPRM Campus. Students will increase awareness on environmental conservation, green engineering and the role of NRCS in promoting sustainable agriculture and farmer’s assistance.
Location and facilities
The flume will be installed at the facilities of the Department of Civil Engineering and Surveying. Field tests will be conducted at the surrounding grass field of the Engineering Complex facility. All the projects will be performed at the University of Puerto Rico at Mayaguez. Figures 4 and 5 show the test site.
Producer Participation Farmer from the region will participate in the demonstration and education program. Plantas de Puerto Rico is a company located in Cayey which will provide advice and species ready to be set inside the experimental flume. A contract will be signed with this company as provider of plants and advisory services during the project duration. Farmers and Plantas de Puerto Rico are eligible for EQIP.
Benefits and Outcomes
1. Retardance classes for the most widely available species recommended for grassed waterways in Puerto Rico and the Caribbean.
2. Determination of the roughness coefficient for Manning’s equation for different combinations of flow depth, flow velocity and vegetation conditions.
3. Updated n-VR design curves for retardance classes (A, B, C, D, E).
4. Recommendations on scour potential for grassed waterways for the selected local species.
5. An Illustrated Guide for Quick Selection of Retardance Index and Manning’s n values for Grassed Waterways
6. A computer software for design of grassed lined waterways, including validated parameters for species in the Caribbean.
7. Recommendations on how to minimize the risk of scour and erosion potential due to stability failure during storm events.
Water management in farms and rural areas rely greatly on grassed waterways. Results from this project will allow USDA-NRCS to provide accurate recommendations to farmers on size and capacity of vegetated drainage channels and waterways. Design of hydrologic/hydraulic modifications in farms and rural areas will be improved. Better design of grass lined waterways, levees, ponds and ditches will reduce soil erosion, greenhouse gases release, improve water quality and mitigate environmental impacts.
The project will increase the knowledge data base on behavior of grass species from Puerto Rico and the Caribbean under water flow conditions in waterways and provide access to new technical tools and guidelines for improved local design criteria.
Technology transfer will be achieved through demonstrations, presentations, videos and the illustrated guideline to a broad community, including farmers, federal and local agencies personnel, professionals, students and faculties from agriculture and environmental fields.
| |