|
|
|
|
1. Project Partner
Joined for a Better Environment
- Puerto Rico Water Resources and Environmental Research Institute
- University of Puerto Rico at Mayagüez
- United States Geological Survey
2. Researchers
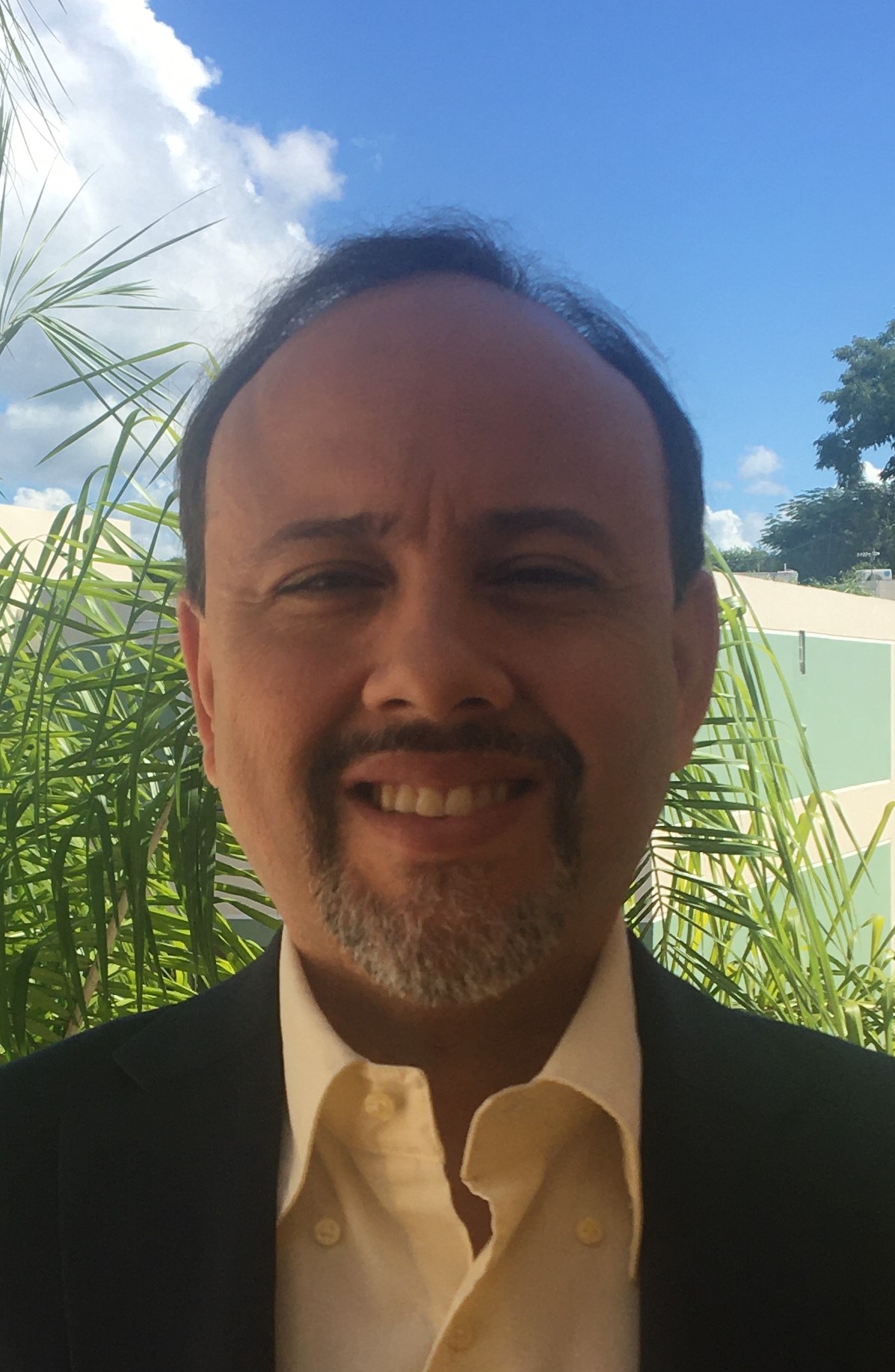
Pedro J, Tarafa Ph.D.
Tenured Professor since July 2020 at
University of Puerto Rico
Mayagüez Campus Pedro.tarafa@upr.edu
3. Students
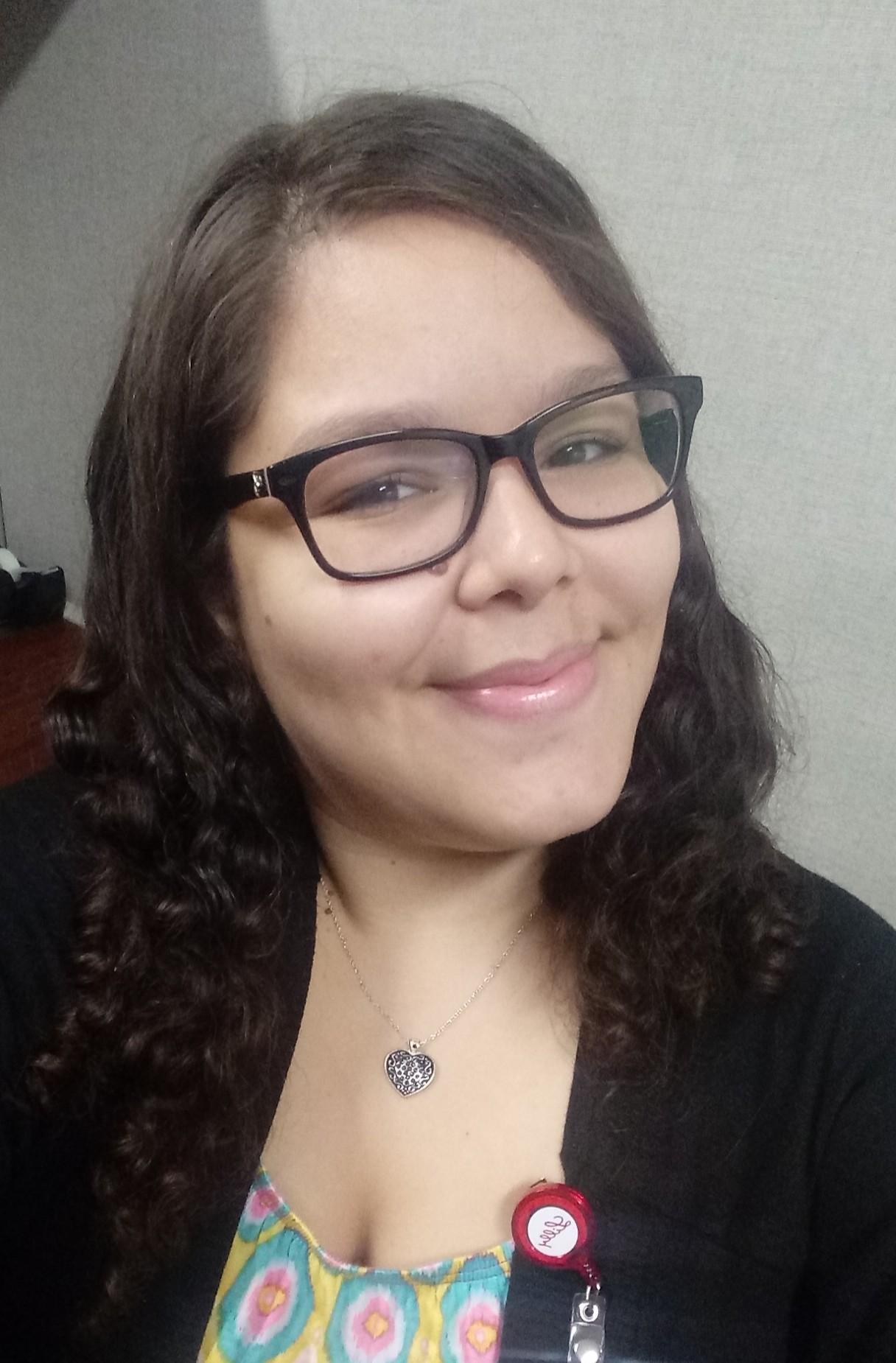
Sulymar Arocho Rodríguez
Bachelor's Degree: Chemical Engineering
Environmental/Water Resources Area
Graduation Date:May 2020
e-mail: sulymar.arocho@upr.edu
Yetzzel Cortés
Concentration: Masters in Engineering and Materials Science
email: yetzzel.cortes@upr.edu<
Sanneri Santiago
Concentration: Chemical Engineering
email: sanneri.santiago@upr.edu<
Emanuel Walker
Concentration: Mechanical Engineering
Alan Aponte
Concentration: Civil Engineering
Estefanía Otero
Concentration: Environmental Sciences
Alba Lacen
University of Puerto Rico at Mayagüez
Graduate studies Civil Engineering
Expected Graduation: May 2021
Carlos Huang
University of Puerto Rico at Mayagüez
Undergraduate: Chemical Engineering
email: carlos.huang@upr.edu<
Austin Dosh
Wester Michigan University
Undergraduate: Chemical Engineering
|
|
|
|
Atrazine and glyphosate monitoring in water streams and degradation using a novel TiO2-based biopolymer
|
Duration: March 1, 2020 to December 31, 2021
Sponsoring Agency: United States Geological Survey (USGS)
Project Strategy, Technical Assistance
and Training Approaches
1. Project Narrative
Pesticides are toxic substances released intentionally into the environment to kill living things.
The increase in the use of pesticides for agriculture activies has resulted in the presence of a
variety of persistent contaminants in surface and ground waters, which compromise the quality of
these water sources. This is particularly true for bodies of water subject to surface runoff and/or
groundwater flow from agricultural sites. Examples of these substances are glyphosate and atrazine.
Both have shown health-related problems, and hence, the US EPA has set maximum contaminant levels (MCL)
to regulate them. Puerto Rico (PR) is not exempt of this situation and as it has several agricultural
sites dedicated for crops and livestock. Previous studies conducted in PR have reported detecting
atrazine in coastal regions and more recent studies ahve detected the presence of atrazine degrading
bacteria in water streams located in Mayagüez area. This proposal aims to start up a database for
atrazine and glyphosate detection, in different streams close to crop sites along the Western region
of PR. These sites could be Finca Perlaborincana in Añasco, Finca Alzamor in Mayag&uulm;, and
the Lajas Experimental Station. The project will consist of establishing a robust, reliable monitoring
protocol and techniques for the detection and quantification of atrazine and glyphosate throughout the
selected sites. We believe this study will serve as a tool to encourage greater awareness of water
quality impaiment for farmers, the scientific community to conduct more research, as well as government
and regulatory agencies along with the public. In addition, we expect to provide support to one graduate
(MS level) and offer training to undergraduate students by enrollment into undergraduate research courses.
Statement of regional or State water problem
Due to their wide use in agricultur, as well as their chemical stability, man-made pesticides have become
ubiquitous pollutants. This is because pesticides are discharged and carried out into the environment mainly
via surface runoff from rural areas and agriculture sites. The presence of pesticide-related contaminants
in water supply sources is an issue that derserves all our attention. This is particularly true for bodies
of water subject to surface runoff and/or groudwater flow from agricultural sites that eventually become
drinking water sources. The increase in production and use of pesticides, for agriculture has resulted in
the presence of a variety of persistent contaminants in surface and ground waters (Santacruz et al., 2005),
which compromise the water quality of these sources (Stone et. al., 2014). Examples of these synthetic
chemical substances are glyphosate and atrazine. Both are well-known and widely used herbicides employed
by farmers to control bradleaf weeds and grasses (Lee et al,. 2002; Seiler et al,.1991). Atrazine is of
particular concern to water supplies due to its popularityu, relatively long half life (60-100 days)
and because it is not strongly absorbed by soil (Koc ~100). In addition, it has been found that atrazine
is a potent endocrine disruptior and has been associated to human reproductive disorders and birth effects
(Prado et al,. 2014). Likewise, glyphosate, along with their additives and surfactants in commercial
formulations, has been found to be toxic in rats (Mal&etilde;cot et al., 2013) and, thus, classified as
carcinogenic to humans (Arroyave et al., 2016). Due to these health-related, problems, the US Environmental
Protection Agency (EPA) has set a maximum contaminant levels (MCLs) of 3 μg/L for protetion from acute
effects and 12 μg/L for protection of chronic effects.
Previous studies conducted in Puerto Rico (PR) by EPA (2006) have reported detecting atrazine in coastal
regions and more recent studies have detected the presence of atrazine degrading bacteria in water streams
located in the Mayagüez area (Sherchan et al., 2013) and atrazine levels from farmland runoffs in
Jobos Bay National Estuarine Research Reserve in Salinas (Williams et al., 2016). PR has agriculural sites
dedicated mostly for human and livestock comsumption, among other activies such as industrial and ornamental
crops. Figure 1 shows a map of the main agricultural sectors in PR. Most of these sites (if not all) are
constantly exposed to pesticides input and, so, to the hauling and draggin of pesticides residues toward
the surface water bodies by water runoffs. Among the most-active crop sites and farms are Finca Agricola
Don Pepe in Arecibo, Finca Perlaborincana in Añasco, Finca Alzamora in Mayagü, Bananera Fabre
in Sabana Grande and the Lajas Experimental Station. All these 5 sites have something in common; they area
located close(between 100 to 2000m) to a water stream (river, creek or canal) and, hence, could have been
causing impairment water quality with respect to pesticides.
Figure 1: Main agriculture sectors in Puerto Rico (source: Department of Agriculture)
Statement of results and benefits
A pest detection program in surface waters will safegaurd agricultural and environmental
resources by ensuring that new introductions of harmful plant pests and diseases are
detected as soon as possible before they have a chance to cause significant damage. A
Strong agricultural pest detection system should involve:
This project will bring the following benefits:
-
A structured, transparent assessment process to identify pest threats
-
Development of monitoring protocols
-
Provide monitoring materials
-
Conduct the pest Monitoring
-
Timely reporting the pest monitoring Results
-
Ensure that the data collected is valid and of high quality
-
Notify of significant pest detections through established protocols
Scientists need to get a better sense of how all that agricultural runoff is affecting water quality. So,
this proposal intents to monitor streams located next or close to crop sites in the western region of PR
to establish a database for traditional parameters such as turbidity, pH, temperature, dissolved, oxygen,
etc., but specifically, for atrazine and glyphosate levels as herbicide contaminant models. To the best of
our knowledge this data, specifically for those two herbicides, is not availabe.
Nature, Scope and Objectives of the project
Nature
Pesticides are toxic substances released intentionally into the environment to kill living things. This includes
substances that kill weeds(herbicides), insects(insecticides), fungus(fungicides), rodents(rodenticides), and others.
The use of toxic pesticides to manage pest problems has become a common practice around the world. Pesticides
are used almost everywhere, not only in agricultural fields, but also in homes, parks, schools, buildings, forests,
and roads. It is difficult to find somewhere where pesticides are not used. In addition, pesticides can be found
in the air we breathe, the food we eat, and the water we drink. To deal with pesticide residues entering our
bodies of water requires creative methods that sustain and regenerate our natural resources. Dr. Tarafa's research
group has been doing work for the development of advanced oxidation processes for the destruction of emergent
contaminants such as atrazine. Hence, this proposal aims to rise up a database for two widely-used herbicides;
atrazine and glyphosate, in different streams close to crop sites along the western region of PR. We believe
this study will serve as a tool to encourage greater awareness of water quality impairment for farmers, the
scientific community to conduct more research, as well as for governments and regulatory agencies along with
the public
Scope
Develop and establish a database for water quality parameters including herbicides such as atrazine and glyphosate
in different waters streams passing near or throughout crop and farm sites in the western region of PR.
Objectives
Select and identify crop and farm sites with water streams to be monitored;
Establish a monitoring protocol and frequency;
Establish and develop appropriate techniques/protocols for the detection/quantification of our target
contaminants(these substances will be atrazine and glyphosate);
Train the people (graduate and undergraduate students) that will b executing the water sampling, monitoring and
analyses.
To accomplish and complete the outlined objectives and goals, it is proposed a one-year project. Table 1 shows the timeline of activities to accomplish the goals of the project within a 12-month period.
Timeline of Activities
Methods, procedures and facilities
Water quality monitoring is important for environmental protection, managing waterways and their catchments,
identifying pollution events and community education. Monitoring consists of making observations and taking
measurements over time that are later analysed and then reported to provide information and knowledge. The
present study will focus on conducting a water quality monitoring at selected water streams that will be
determined according to their location and proximity to farms or crop sites. For this proposal the water
quality monitoring plan will include assessing traditional physical, chemical, and bacteriological
parameters such as turbidity, pH, temperature, dissolved oxygen, nitrogen and phosphorus and faecal
coliforms. In addition, it is intended to evaluate the presence, and levels, of pesticide pollutants
using atrazine and glyphosate as herbicide contaminant models. The plan for this water quality monitoring
will consists in developing and executing the following phases:
The following methodology will be followed:
-
Monitoring plan- The monitoring plan will describe the monitoring strategy to be used. It will ba
developed before starting a monitoring project. The monitoring plan will provide a guide for why,
how, when, and where to monitor the water quality parameters. The monitroring plan will help maintian
consistency in the water sample collection, handling and analysis.
-
Selecting the sites- Selecting the appropriate sites for monitoring water quality will depend option
the objective already stated for this proposal. However, there will be geographic scales to consider
in selecting the appropriate monitoring site. For example, does the sample point provide represetative
data at that spot? In addition to the scientific considerations for monitoring sites (i.e using standart
data gathering techniques for consistency, maintaining data quality, etc), there will also be practical
considerations. For example, easy access with landowner permission. Sampling stations should be also
accessible for all flow considerations that will be sampled.
- For this study, we will be considering sampling points for water streams passing nearby crop sites
in the Western region of PR. THese sites could be Finca Perlaborincana in Añasco, Finca
Alzamora in Mayag&uulm;ez, and the Lajas Experimental Station.
-
Methods for atrazine and glyphosate detection- Both attrazine and glyphosate will be quantified
by means of gas chromatography with flame ionization detector (GC-FID) and high-performance liquid
chromatrography as describes elsewhere (Avil&etilde;s et al., 2017; Wang et al., 2016)
-
Water sampling and analysis- All samples will be collected, handled, processed, and analysed
according to the USGS "Handbooks for Water-Resources Investigations" Chapters A4 and A5 (Wilde and
Radtke, 2006)
- Water samples will be collected in duplicate for each selected site in a weekly basis for 8 months.
Each sample will be taken in sterile whirlpack bags or polyproplyne bottles and will be kept on
ice and processed within 6 hours.
-
Data Quality and Management- The goal of data gathering is to produce data of a known quality which is
adequate for the intented use. Hence, appropriate methodology will be used to eliminate flaws and errors
before they compromise the quality of data collected. To assure that the data are credible, procedures
must be documented, and regular evaluations of precision and accuracy should be conducted.
- To facilitate the collection, storage, and eventual archiving of the data, an external drive with a 3TB
capacity will be purchased. The data will be stored in standard, non-proprietary file formats, such as
PDF, ASCII, TIFF or JPEG2000, to facilitate both data sharing and long-time preservation. A detailed
data description and experimental conditions will be included in an accompanying text file and/or
laboratory notebook description.
Additionally, data will be redudantly sotred ad the UPRM document management system (DMS). The
Graduate Student's Computer lab provides dedicated scanners to scan laboratory notebooks. Their PDF
files will accompany the data and originals and will be secured by the PI. Physical samples will be
labelled and soted in a designated area of the laboratory. Upon request, access to the data will be
provided via contact with PI.
Environmental Engineering Laboratory (EEL): The EEL is located in the deparment of Civil Engineering
and Surveying at the University of Puerto Rico at Mayag&uulm;ez (UPRM). It provides academic services
to train and develop skilled students, professionals, and other members of the academic community in
environmental science and engineering. The EEL is used for formal and informal courses, research
activites, and community services. The facilities include 3,430 ft² of indoor laboratory space
and 1,721 ft² of field space. The facilities are well equipped with instrumentation for water,
air, waste, and soil analysis. The field site, which is located next to the Civil Engineering building,
containts unsaturated and saturated wells and instrumentation and climotologic station. It also
supports geophysical and underground field measurements. The facilities also house the EnviroBeds
Facilities, which consist of several subsurface and engineering-environmental-systems physical models,
and computational facilities. These facilities permit students to get acquainted and physical models, and
computational facilities. These facilities permit students to get acquianted and use the most advanced
technology for water resource management and pollution control. It has alos instrumentation for analytical
analysis such as HPLC, GC, TOC and IC.
Related Research
Glyphosate (N-[phosphonmethyl]glycine) is a postemergence, broad-spectrum, and non-selective herbicide
that exhibits excellent performance and effect in weed control (Lee et al., 2002). Due to its relatively
low toxicity to mammals, glyphosate has become the most frequently used herbicide all around the world
(Guo et al., 2014). However, the indiscriminate application of glyphosate may easily lead to high-level
residues in agricultural products, which can create a potential threat to human health (Wei et al., 2013).
The median lethal dose (LD50) of glyphosate in rats is around 5000 mg kg—1 (Malécot et al., 2013).
Therefore, it is of a significant importance to establish a reliable method for the determination of glyphosate
in agricultural samples.
Glyphosate, being a phosphoric amino derivative of glycine, tends to behave like the inorganic phosphates
naturally present in the soil and as such is generally persistent. The reported Biochemical Oxygen demand
(BOD) and Chemical Oxygen Demand (COD) values are less than 2 mg/g and 0.53 g/g respectively, thus the
molecule cannot be considered easily biodegradable. Also considering the glyphosate density of about 1.7 g/cm³
and its limited volatility, it is fairly straightforward to deduce that it will be difficult for the substance
to evaporate or to remain suspended in the air for a long time after its application.
Glyphosate is a strong chelating agent. Thus, it creates, through physical chemical links, the complexes that
immobilize the mineral micronutrients of the soil (calcium, iron, magnesium, manganese, nickel, zinc, etc.)
making them unavailable to plants (Glass, 1984). The elimination of glyphosate from the environment through
physical processes is very limited. Microbial degradation can significantly eliminate glyphosate from the soil,
which can be performed in the presence or absence of oxygen and can vary greatly according to the characteristics
of the soil and the pH range at which the reaction takes place (Williams et al., 2000).
The time, estimated in the laboratory, necessary to degrade 50% of glyphosate present in the water is less
than 14 days in aerobic conditions and about 14–22 days in anaerobic conditions. While for the glyphosate
present in the soil the degradation time in aerobic conditions is about 2–3 days (estimated time in laboratory tests)
(Williams et al., 2000). Biological degradation and associated times are often described and calculated using
the models and equations related to first-order kinetics. The degradation of the herbicide molecule can
follow two paths; the first is based on the breakdown of the carbon-nitrogen bond and leads to the formation
of Aminomethylphosphonic acid (AMPA, main metabolite of glyphosate), while the second way is based on the
splitting of the carbon- phosphorus bond, resulting in the formation of sarcosine and glycine (Battaglin et al., 2014).
In general, the total concentration of glyphosate in the ground varies from 4 to 180 days, making it therefore a
potentially contaminating substance for soil and likely for groundwater as well (Borggaard and Gimsing, 2008;
Vereecken, 2005). Numerous laboratory studies have shown that the absorption constant of the molecule in the
soil varies between 8 and 377 dm3/kg, depending on the characteristics and composition of the soil (clay, sand,
or gravel). This coefficient value indicates a high absorption in the soil. In water, the half-life of
glyphosate varies from a few days to 91 days (Borggaard and Gimsing, 2008; Vereecken, 2005).
On the other hand, atrazine (2-chloro-4-ethylamine-6-isopropylamino-1, 3, 5 triazine), a member of s-triazine
herbicide family, is designed to inhibit photosystem-II in plants and is widely used to control broad-leaf weeds
in agricultural fields, golf courses and in residential lawns (Devers et al., 2004). Atrazine can persist in coastal
waters for more than a year and has been detected in estuaries and coastal environments in many parts of the world
(Pereira and Hostetler, 1993; Brodie et al., 2012). The endocrine disrupting properties of atrazine in mammals,
amphibians and fishes are well documented (Hayes et al., 2002, 2011) and high doses are believed to be carcinogenic
to mammals (Gammon et al., 2005). In addition, research have indicated that atrazine can have a disruptive impact
on marine communities including coral reefs (Cantin et al., 2007), phototrophic bacteria, algae (Magnusson et al., 2008),
mangroves (Bell and Duke, 2005) and sea grasses (Haynes et al., 2000). Coral reefs and mangroves represent a valuable
economic resource to communities in the United States and the Caribbean Islands. Therefore, there is a need to evaluate
the presence and impact of herbicides such as atrazine in water systems.
Training Potential
From this research proposal we expect to provide training and support for one graduate student (MS level) and two to
four undergraduate students. In addition, more undergraduate students can be incorporated by enrollment in undergraduate
research courses. All students will have access to the necessary instrumentation and laboratory facilities affiliated
to the project. The participants will receive proper laboratory safety training and additional instruction with
ancillary workshops. Students will participate in the dissemination of the project findings by presentations
in local and regional conferences. To this purpose they will receive training on oral presentations and poster preparations.
Finally, they will receive immediate feedback by their supervisors and their peers on the development of the project,
incoming tasks and goals.
References
-
Arroyave, J. M., Waiman, C. C., Zanini, G. P., & Avena, M. J. (2016). Effect of humic acid on the
adsorption/desorption behavior of glyphosate on goethite. Isotherms and kinetics. Chemosphere, 145, 34-41.
-
Avilés, A., Hernandez, C., Ambrose, J., Suárez, O. M., & Tarafa, P. J. (2017). Degradation of atrazine
with titanium dioxide immobilised in compact recycled glass. Journal of Environmental Engineering and Science, 12(4) 79-85.
-
Battaglin, W. A., Meyer, M. T., Kuivila, K. M., & Dietze, J. E. (2014). Glyphosate and its degradation
product AMPA occur frequently and widely in US soils, surface water, groundwater, and precipitation. JAWRA Journal of the American Water Resources Association, 50(2), 275-290.
-
Bell, A. M., & Duke, N. C. (2005). Effects of Photosystem II inhibiting herbicides on mangroves—
preliminary toxicology trials. Marine Pollution Bulletin, 51(1-4), 297-307.
-
Borggaard, O. K., & Gimsing, A. L. (2008). Fate of glyphosate in soil and the possibility of leaching to
ground and surface waters: a review. Pest management science, 64(4), 441-456.
-
Cantin, N. E., Negri, A. P., & Willis, B. L. (2007). Photoinhibition from chronic herbicide exposure
reduces reproductive output of reef-building corals. Marine Ecology Progress Series, 344, 81-93.
-
Devers, M., Soulas, G., & Martin-Laurent, F. (2004). Real-time reverse transcription PCR analysis of
expression of atrazine catabolism genes in two bacterial strains isolated from soil. Journal of microbiological methods, 56(1), 3-15.
-
EPA, 2006. Decision Documents for atrazine.
https://www3.epa.gov/pesticides/chem_search/reg_actions/reregistration/red_PC-080803_1-Apr-06.pdf.
-
Gammon, D. W., Aldous, C. N., Carr Jr, W. C., Sanborn, J. R., & Pfeifer, K. F. (2005). A risk assessment
of atrazine use in California: human health and ecological aspects. Pest Management Science: formerly Pesticide Science, 61(4), 331-355.
-
Glass, R. L. (1984). Metal complex formation by glyphosate. Journal of Agricultural and Food
Chemistry, 32(6), 1249-1253.
-
Guo, J., Zhang, Y., Luo, Y., Shen, F., & Sun, C. (2014). Efficient fluorescence resonance energy transfer
between oppositely charged CdTe quantum dots and gold nanoparticles for turn-on fluorescence detection of glyphosate. Talanta, 125, 385-392.
-
Hayes, T. B., Collins, A., Lee, M., Mendoza, M., Noriega, N., Stuart, A. A., & Vonk, A. (2002).
Hermaphroditic, demasculinized frogs after exposure to the herbicide atrazine at low ecologically relevant doses. Proceedings of the National Academy of Sciences, 99(8), 5476-5480.
-
Hayes, T. B., Anderson, L. L., Beasley, V. R., De Solla, S. R., Iguchi, T., Ingraham, H., & Luque, E. H.
(2011). Demasculinization and feminization of male gonads by atrazine: consistent effects across vertebrate classes. The Journal of steroid biochemistry and molecular biology, 127(1-2), 64-73.
-
Haynes, D., Müller, J., & Carter, S. (2000). Pesticide and herbicide residues in sediments and
seagrasses from the Great Barrier Reef World Heritage Area and Queensland coast. Marine Pollution Bulletin, 41(7-12), 279-287.
-
Lee, E., Zimmerman, L., Bhullar, B., Thurman, E. (2002). Linker-assisted immunoassay and liquid
chromatography/massspectrometry for the analysis of glyphosate, Anal. Chem.74, 4937–4943.
-
Magnusson, M., Heimann, K., & Negri, A. P. (2008). Comparative effects of herbicides on
photosynthesis and growth of tropical estuarine microalgae. Marine Pollution Bulletin, 56(9), 1545-1552.
-
Malécot, M., Guével, B., Pineau, C., Holbech, B. F., Bormans, M., & Wiegand, C. (2013). Specific
proteomic response of Unio pictorum mussel to a mixture of glyphosate and microcystin-LR. Journal of proteome research, 12(11), 5281-5292.
-
Pereira, W. E., & Hostettler, F. D. (1993). Nonpoint source contamination of the Mississippi River and
its tributaries by herbicides. Environmental science & technology, 27(8), 1542-1552.
-
Prado, B., Duwig, C., Hidalgo, C., Müller, K., Mora, L., Raymundo, E., and Etchevers, J. D. (2014).
Transport, sorption and degradation of atrazine in two clay soils from Mexico: Andosol and Vertisol, Geoderma, 232–234, pp. 628–639.
-
Santacruz, G., Bandala, E. R., and Torres, L. G. (2005). Chlorinated pesticides (2,4-D and DDT)
biodegradation at high concentrations using immobilized Pseudomonas fluorescens. J Environ Sci Health Part B-Pesticides Food Contaminants and Agricultural Wastes, 40, 571–583.
-
Seiler, A., Brenneisen, P., and Green, D. H. (1991). Benefits and Risks of Plant-Protection Products –
Possibilities of Protecting Drinking-Water – Case Atrazine. In: Rapinat, M. (Ed.). Blackwell Scientific Publ, Florence, Italy, pp. 31–42.
-
Sherchan, S. P., Bachoon, D. S., Otero, E., & Ramsubhag, A. (2013). Molecular detection of atrazine
catabolism gene atzA in coastal waters of Georgia, Puerto Rico and Trinidad. Marine pollution bulletin, 69(1-2), 215-218.
-
Stone, W. W., Gilliom, R. J., and Martin, J. D., 2014. An Overview Comparing Results from Two
Decades of Monitoring for Pesticides in the Nation’s Streams and Rivers, 1992–2001 and 2002–2011, , U.S. Geological Survey Scientific Investigations Report 2014–5154, 23 p., http://dx.doi.org/10.3133/sir20145154.
-
Vereecken, H. (2005). Mobility and leaching of glyphosate: a review. Pest management science,
61(12), 1139-1151.
-
Wang, L., Bi, Y., Hou, J., Li, H., Xu, Y., Wang, B., ... & Ding, L. (2016). Facile, green and clean one-step
synthesis of carbon dots from wool: Application as a sensor for glyphosate detection based on the inner filter effect. Talanta, 160, 268-275.
-
Wei, X., Gao, X., Zhao, L., Peng, X., Zhou, L., Wang, J., & Pu, Q. (2013). Fast and interference-free
determination of glyphosate and glufosinate residues through electrophoresis in disposable microfluidic chips. Journal of Chromatography A, 1281, 148-154.
-
Wilde, F. D., & Radtke, D. B. (Eds.). (2006). Handbooks for Water-resources Investigations: National
field manual for the collection of water-quality data. Field measurements. US Department of the Interior, US Geological Survey.
-
Williams, C. O., Lowrance, R., Potter, T., Bosch, D. D., & Strickland, T. (2016). Atrazine Transport
Within a Coastal Zone in Southeastern Puerto Rico: a Sensitivity Analysis of an Agricultural Field Model and Riparian Zone Management Model. Environmental Modeling & Assessment, 21(6), 751-761.
-
Williams, G. M., Kroes, R., & Munro, I. C. (2000). Safety evaluation and risk assessment of the
herbicide Roundup and its active ingredient, glyphosate, for humans. Regulatory toxicology and pharmacology, 31(2), 117-165.
|
|